What are quantum coherence and decoherence?
Quantum coherence and decoherence are fundamental indications of how well a system of quantum objects -- atoms or other quantum particles -- maintains its relationships and how reliably the ongoing operation of that quantum system can be predicted over time.
Coherence
Quantum coherence is the ability of a quantum system of objects to exist in superposition, or simultaneously in multiple places or states, until measured.
Quantum theory, or quantum mechanics, presents a quantum system's behavior through mathematical relationships, often plotted visually as a waveform. When a system of quantum objects is coherent, the relationship or influence between the quantum objects produces a discernable pattern. The longer quantum coherence remains, the more time that quantum system has for its work, including quantum computing using qubits, quantum sensing or quantum cryptography.
Decoherence
Decoherence occurs when a quantum system transitions from superposition -- the ability to exist in multiple states simultaneously -- to a measurable, classical state, such as a binary state, either 1 or 0, for instance.
Due to their fragility, quantum objects and their relationships are challenging to sustain. Other objects and fields of influence -- foreign materials, photons from laser beams or energy from microwaves -- disturb and alter the relationship between quantum objects, which is often unpredictable or undecipherable. The underlying relationship between the quantum objects becomes decoherent. When plotted visually, the now-disturbed waveform -- representing the quantum relationship -- appears erratic or random. Any information represented by that system of quantum objects is lost.
Concepts of coherence
Coherence is the consistent, repeatable behaviors of particles or waves as they interact, and decoherence is the loss of those reliable behaviors.
Quantum mechanics focuses on waves. Fortunately, there are practical, everyday examples of waves and wave interactions to help clarify coherence, including ocean waves, sound waves, and compression and expansion waves, such as a Slinky descending a staircase. Likewise, quantum objects feature photons or light waves, electromagnetic waves such as radio waves or microwaves, as well as matter waves including vectors of atoms and electrons.
Waves inevitably interact in space and time, adding and subtracting energy from each other, resulting in a distinct wave with new characteristics from that quantum interference.
For instance, the image below shows the interaction between waves that can lead to two distinct occurrences -- constructive interference and destructive interference.
- Consider two waves of equal amplitude and phase, or occurring in synchronization at the same time. Those waves join, producing a single wave of greater amplitude than the original one. This is constructive interference.
- Next, consider two waves of equal amplitude that are precisely out of phase or occurring at exactly the opposite time from one another. Those waves negate each other. The result is no wave -- a flat line. This is destructive interference.
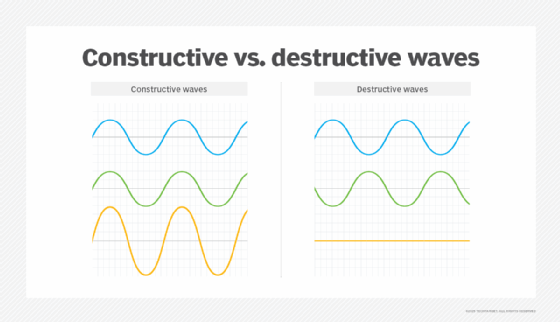
- Finally, consider two waves of differing amplitudes and phases. Such hybrid wave interactions pair constructive and destructive behaviors to produce a complex periodic wave. This hybrid combines the two original waves into sometimes odd new patterns -- often with cumbersome mathematical representations.
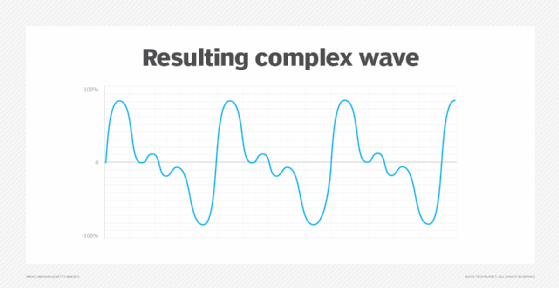
The primary concerns are the coherence of the resulting waves -- regardless of their shape -- and maintaining a predictable relationship and behavior over time.
Although this discussion originates in classical physics, such as the relationship between sound waves, the analysis applies to quantum objects and their exhibited matter waves. Sometimes, when quantum objects interact, their waves combine to create complex hybrid waves. If the relationship between quantum objects is sustained, the quantum objects and the information they represent maintain coherence.
However, quantum objects are sensitive to any environmental influences. When a quantum object encounters any influence, that object and the relationship between affected quantum objects are changed, sometimes profoundly altering the wave patterns exhibited by the quantum objects. This typically leads to a loss of any consistent or repeatable wave pattern. The behavior becomes decoherent.
Decoherence is a fundamental, persistent problem in quantum mechanics because quantum objects are easily disrupted. For example, a decoherent qubit causes errors in quantum computing. Quantum scientists and engineers invest significant effort in maintaining coherence and preventing decoherence.
Examples of quantum coherence and decoherence
Among the examples of quantum coherence and decoherence, here are two that can aid in understanding these behaviors:
- Quantum coherence is similar to a spinning coin; it's neither heads nor tails -- or both heads and tails -- in its free-spinning state. Quantum decoherence occurs when gravity and the table's surface interfere with the spinning coin, which enters a classical state of either heads or tails after landing.
- In a quantum computing example, a qubit must remain coherent and maintain superposition to perform complex calculations. When the qubit experiences environmental noise or other interference, it's likely to lose superposition and collapse into a single classical state that is undesirable for quantum computing.
Still, decoherence is not always undesirable. At times, it's a goal of devices such as quantum sensors. By sending coherent quantum objects or waves into an unknown environment, the resulting patterns and outcomes of the decoherence provide helpful information about the unknown environment.
For instance, radar uses electromagnetic signals reflected from an object to provide helpful information about the position and speed of that object. Quantum sensors operate on the same broad principle, but their incredible sensitivity delivers far greater detail about the object. The same is true for medical imaging, from X-rays to MRIs. Quantum medical imaging devices promise more detailed medical examinations and diagnoses than traditional imaging systems.